Plotted on a graph, the pattern’s “hits” look like this:
In theory, those smaller side peaks around the main one should be symmetrical. In practice, we’re dealing with probabilities and individual bits of light, so the result usually deviates a bit from the ideal. Anyway, the big question here is: why this pattern?
Turns out, it’s exactly what we’d expect if light is made of waves, not particles. Waves collide and interfere with each other, causing ripples. If you toss two pebbles into a pond at the same time, the waves produced by each meet each other and produce places of
higher-than-normal or lower-than-normal water-rises. Some waves reinforce each other or, if one’s crest meets another’s trough, they cancel out at that spot.
So this early-twentieth-century result of an interference pattern, which can only be caused by waves, showed physicists that light is a wave or at least acts that way when this experiment is performed. The fascinating thing is that when solid physical bodies like electrons were used, they got exactly the same result. Solid particles have a wave nature too! So, right from the get-go, the double-slit experiment yielded amazing information about the nature of reality. Solid objects have a wave nature!
Unfortunately, or fortunately, this was just the appetizer. Few realized that true strangeness was only beginning.
The first oddity happens when just one just photon or electron is allowed to fly through the apparatus at a time. After enough have gone through and been individually detected, this same interference pattern emerges. But how can this be?
With what
is each of those electrons or photons interfering? How can we get an interference pattern when there’s only indivisible object in there at a time?
A single photon hits the detector.
A second photon hits the detector.
A third photon hits the detector.
Somehow, these individual photons add up to an interference pattern!
There has never been a truly satisfactory answer for this. Wild ideas keep emerging. Could there be other electrons or photons “next door” in a parallel universe, from another experimenter doing the same thing? Could their electrons be interfering with ours? That’s so far-fetched that few believe it.
The usual interpretation of why we see an interference pattern is that photons or electrons have two choices when they encounter the double slit. They do not actually exist as real entities in real places until they are observed, and they aren’t observed until they hit the final detection barrier. So when they reach the slits, they exercise their probabilistic freedom of taking
both
choices. Even though
actual
electrons or photons are indivisible, and never split themselves under any conditions whatsoever, their existence as
probability waves
are another story. Thus, what go “through the slit” are not actual entities but just probabilities.
The probability waves of the individual photons interfere with themselves!
When enough have gone through, we see the overall interference pattern as all probabilities congeal into actual entities making impacts and being observed—as waves.
Sure it’s weird, but this, apparently, is how reality works. And this is just the very beginning of quantum weirdness. Quantum theory, as we mentioned in the last chapter, has a principle called complementarity, which says that we can observe objects to be one thing or another—or have one position or property or another, but never both. It depends on what one is looking for and what measuring equipment is used.
Now, suppose we wish to know which slit a given electron or photon has gone through on its way to the barrier. It’s a fair enough question, and it’s easy enough to find out. We can use polarized light (that is, light whose waves vibrate either horizontally or vertically or else slowly rotate their orientation) and when such a mixture is used, we get the same result as before. But now let’s determine which slit each photon is going through. Many different things have been used, but in this experiment we’ll use a “quarter wave plate” or QWP in front of each slit. Each quarter wave plate alters the polarity of the light in a specific way. The detector can let us know the polarity of the incoming photon. So by noting the polarity of the photon when it’s detected, we know which slit it went through.
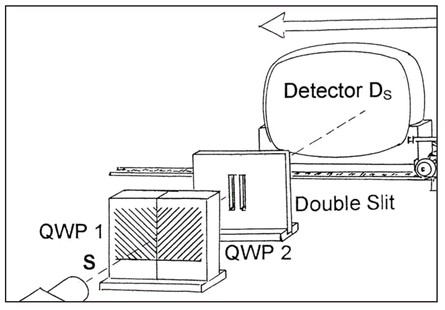
Now we repeat the experiment, shooting photons through the slits one at a time, except this time we know which slot each photon goes through. Now the
results
dramatically change. Even though QWPs do not alter photons other than harmlessly shifting their polarities (later, we prove that this change in results is not caused by the QWPs), now we no longer get the interference pattern. Now the curve suddenly changes to what we’d expect if the photons were particles:
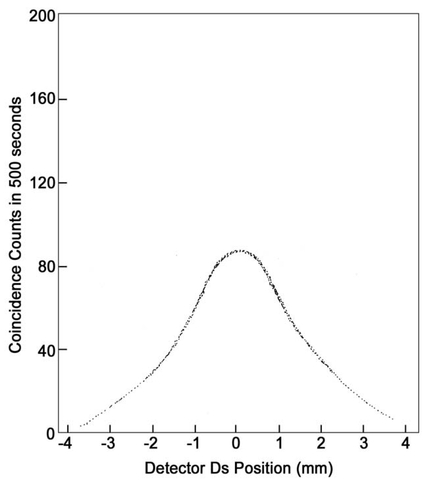
Something’s happened. It turns out that the mere act of measurement, of learning the path of each photon, destroyed the photon’s freedom to remain blurry and undefined and take both paths until it reached the barriers. Its “wave-function” must have collapsed at our measuring device, the QWPs, as it instantly “chose” to become a particle and go through one slit or the other. Its wave nature was lost as soon as it lost its blurry probabilistic not-quite-real state. But why should the photon have chosen to collapse its wave-function? How did it
know
that we, the observer, could learn which slit it went through?
Countless attempts to get around this, by the greatest minds of the past century, have all failed. Our
knowledge
of the photon or electron path alone caused it to become a definite entity ahead of the previous time. Of course, physicists also wondered whether this bizarre behavior might be caused by some interaction between the which-way QWP detector or various other devices that have been tried, and the photon. But no. Totally different which-way detectors have been built, none of which in any way disturb the photon, yet we always lose the interference pattern. The bottom line conclusion, reached after many years, is that it’s simply not possible to gain which-way information
and
the interference pattern caused by energy waves.
We’re back to quantum theory’s complementarity—that you can measure and learn just one of a pair of characteristics but never both at the same time. If you fully learn about one, you will know nothing about the other. And, just in case you’re suspicious of the quarter wave plates, let it be said that when used in all other contexts, including double-slit experiments but without information-providing polarization-detecting barriers at the end, the mere act of changing a photon’s polarization never has the slightest effect on the creation of an interference pattern.
Okay, let’s try something else. In nature, as we saw in the last chapter, there are entangled particles or bits of light (or matter) that were born together and therefore share a wave-function according to quantum theory. They can fly apart—even across the width of the galaxy—and yet they still retain this connection, this knowledge of each other. If one is meddled with in any way so that it
loses its “anything’s possible” nature and has to decide instantly to materialize with, say, a vertical polarization, its twin will then instantaneously materialize too, and with a horizontal polarity. If one becomes an electron with an up spin, the twin will too, but with a down spin. They’re eternally linked in a complementary way.