Millions of years later, and after more splitting events, one of the descendant dinosaur species, node Y, itself split into two more species, one eventually producing all the bipedal, carnivorous dinosaurs and the other producing all living birds. This critical moment in evolutionary history—the birth of the ancestor of all birds—wouldn’t have looked so dramatic at the time. We wouldn’t have seen the sudden appearance of flying creatures from reptiles, but merely two slightly different populations of the same dinosaur, probably no more different than members of diverse human populations are today. All the important change occurred thousands of generations after the split, when selection acted on one lineage to promote flight and on the other to promote the traits of bipedal dinosaurs. It is only in retrospect that we can identify species Y as the common ancestor of
T. rex
and birds. These evolutionary events were slow, and seem momentous only when we arrange in sequence all the descendants of these diverging evolutionary streams.
But species don’t
have
to split. Whether they do depends, as we’ll see, on whether circumstances allow populations to evolve enough differences that they are no longer able to interbreed. The vast majority of species—more than 99 percent of them—go extinct without leaving any descendants. Others, like gingko trees, live millions of years without producing many new species. Speciation doesn’t happen very often. But each time one species splits into two, it doubles the number of opportunities for
future
speciation, so the number of species can rise exponentially. Although speciation is slow, it happens sufficiently often, over such long periods of history, that it can easily explain the stunning diversity of living plants and animals on earth.
Speciation was so important to Darwin that he made it the title of his most famous book. And that book did give some evidence for the splitting. The only diagram in the whole of
The Origin
is a hypothetical evolutionary tree resembling figure 1. But it turns out that Darwin didn’t really explain how new species arose, for, lacking any knowledge of genetics, he never really understood that explaining species means explaining barriers to gene exchange. Real understanding of how speciation occurs began only in the 1930s. I’ll have more to say about this process, which is my own area of research, in chapter 7.
It stands to reason that if the history of life forms a tree, with all species originating from a single trunk, then one can find a common origin for every pair of twigs (existing species) by tracing each twig back through its branches until they intersect at the branch they have in common. This node, as we’ve seen, is their common ancestor. And if life began with one species and split into millions of descendant species through a branching process, it follows that every pair of species shares a common ancestor sometime in the past. Closely related species, like closely related people, had a common ancestor that lived fairly recently, while the common ancestor of more distantly related species, like that of distant human relatives, lived further back in the past. Thus, the idea of
common ancestry—
the fourth tenet of Darwinism—is the flip side of speciation. It simply means that we can always look back in time, using either DNA sequences or fossils, and find descendants joining at their ancestors.
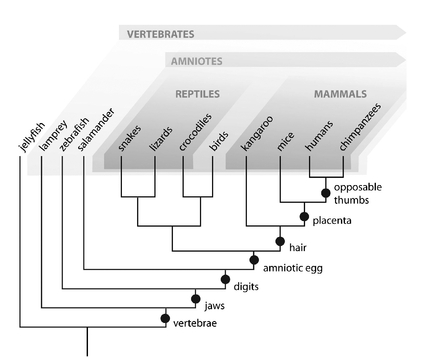
FIGURE 2
. A phylogeny (evolutionary tree) of vertebrates, showing how evolution produces a hierarchical grouping of features, and thus of species containing these features. The dots indicate where on the tree each trait arose.
Let’s examine one evolutionary tree, that of vertebrates (figure 2). On this tree I’ve put some of the features that biologists use to deduce evolutionary relationships. For a start, fish, amphibians, mammals, and reptiles all have a backbone—they are “vertebrates”—so they must have descended from a common ancestor that also had vertebrae. But within vertebrates, reptiles and mammals are united (and distinguished from fish and amphibians) by having an “amniotic egg”—the embryo is surrounded by a fluid-filled membrane called the amnion. So reptiles and mammals must have had a more recent common ancestor that itself possessed such an egg. But this group also contains two subgroups, one with species that all have hair, are warm-blooded, and produce milk (that is, mammals), and another with species that are cold-blooded, scaly, and produce watertight eggs (that is, reptiles). Like all species, these form a nested hierarchy: a hierarchy in which big groups of species whose members share a few traits are subdivided into smaller groups of species sharing more traits, and so on down to species, like black bears and grizzly bears, that share nearly all their traits.
Actually, the nested arrangement of life was recognized long before Darwin. Starting with the Swedish botanist Carl Linnaeus in 1635, biologists began classifying animals and plants, discovering that they consistently fell into what was called a “natural” classification. Strikingly, different biologists came up with nearly identical groupings. This means that these groupings are not subjective artifacts of a human need to classify, but tell us something real and fundamental about nature. But nobody knew what that something was until Darwin came along and showed that the nested arrangement of life is precisely what evolution predicts. Creatures with recent common ancestors share many traits, while those whose common ancestors lay in the distant past are more dissimilar. The “natural” classification is itself strong evidence for evolution.
Why? Because we don’t see such a nested arrangement if we’re trying to arrange objects that haven’t arisen by an evolutionary process of splitting and descent. Take cardboard books of matches, which I used to collect. They don’t fall into a natural classification in the same way as living species. You could, for example, sort matchbooks hierarchically beginning with size, and then by country within size, color within country, and so on. Or you could start with the type of product advertised, sorting thereafter by color and then by date. There are many ways to order them, and everyone will do it differently. There is no sorting system that all collectors agree on. This is because rather than evolving, so that each matchbook gives rise to another that is only slightly different, each design was created from scratch by human whim.
Matchbooks resemble the kinds of creatures expected under a creationist explanation of life. In such a case, organisms would not have common ancestry, but would simply result from an instantaneous creation of forms designed de novo to fit their environments. Under this scenario, we wouldn’t expect to see species falling into a nested hierarchy of forms that is recognized by all biologists.
2
Until about thirty years ago, biologists used visible features like anatomy and mode of reproduction to reconstruct the ancestry of living species. This was based on the reasonable assumption that organisms with similar features also have similar genes, and thus are more closely related. But now we have a powerful, new, and independent way to establish ancestry: we can look directly at the genes themselves. By sequencing the DNA of various species and measuring how similar these sequences are, we can reconstruct their evolutionary relationships. This is done by making the entirely reasonable assumption that species having more similar DNA are more closely related—that is, their common ancestors lived more recently. These molecular methods have not produced much change in the pre-DNA era trees of life: both the visible traits of organisms and their DNA sequences usually give the same information about evolutionary relationships.
The idea of common ancestry leads naturally to powerful and testable predictions about evolution. If we see that birds and reptiles group together based on their features and DNA sequences, we can predict that we should find common ancestors of birds and reptiles in the fossil record. Such predictions have been fulfilled, giving some of the strongest evidence for evolution. We’ll meet some of these ancestors in the next chapter.
The fifth part of evolutionary theory is what Darwin clearly saw as his greatest intellectual achievement: the idea of
natural selection.
This idea was not in fact unique to Darwin—his contemporary, the naturalist Alfred Russel Wallace, came up with it at about the same time, leading to one of the most famous simultaneous discoveries in the history of science. Darwin, however, gets the lion’s share of credit because in
The Origin
he worked out the idea of selection in great detail, gave evidence for it, and explored its many consequences.
But natural selection was also the part of evolutionary theory considered most revolutionary in Darwin’s time, and it is still unsettling to many. Selection is both revolutionary and disturbing for the same reason: it explains apparent design in nature by a purely materialistic process that doesn’t require creation or guidance by supernatural forces.
The idea of natural selection is not hard to grasp. If individuals within a species differ genetically from one another, and some of those differences affect an individual’s ability to survive and reproduce in its environment, then in the next generation the “good” genes that lead to higher survival and reproduction will have relatively more copies than the “not so good” genes. Over time, the population will gradually become more and more suited to its environment as helpful mutations arise and spread through the population, while deleterious ones are weeded out. Ultimately, this process produces organisms that are well adapted to their habitats and way of life.
Here’s a simple example. The wooly mammoth inhabited the northern parts of Eurasia and North America, and was adapted to the cold by bearing a thick coat of hair (entire frozen specimens have been found buried in the tundra).
3
It probably descended from mammoth ancestors that had little hair—like modern elephants. Mutations in the ancestral species led to some individual mammoths-like some modern humans—being hairier than others. When the climate became cold, or the species spread into more northerly regions, the hirsute individuals were better able to tolerate their frigid surroundings, and left more offspring than their balder counterparts. This enriched the population in genes for hairiness. In the next generation, the average mammoth would be a bit hairier than before. Let this process continue over some thousands of generations, and your smooth mammoth gets replaced by a shaggy one. And let many different features affect your resistance to cold (for example, body size, amount of fat, and so on), and those features will change concurrently.
The process is remarkably simple. It requires only that individuals of a species vary genetically in their ability to survive and reproduce in their environment. Given this, natural selection—and evolution—are inevitable. As we shall see, this requirement is met in every species that has ever been examined. And since many traits can affect an individual’s adaptation to its environment (its “fitness”), natural selection can, over eons, sculpt an animal or plant into something that looks designed.
It’s important to realize, though, that there’s a real difference in what you’d expect to see if organisms were consciously designed rather than if they evolved by natural selection. Natural selection is not a master engineer, but a tinkerer. It doesn’t produce the absolute perfection achievable by a designer starting from scratch, but merely the best it can do with what it has to work with. Mutations for a perfect design may not arise because they are simply too rare. The African rhinoceros, with its two tandemly placed horns, may be better adapted at defending itself and sparring with its brethren than is the Indian rhino, graced with but a single horn (actually, these are not true horns, but compacted hairs). But a mutation producing two horns may simply not have arisen among Indian rhinos. Still, one horn is better than no horns. The Indian rhino is better off than its hornless ancestor, but accidents of genetic history may have led to a less than perfect “design.” And, of course, every instance of a plant or animal that is parasitized or diseased represents a failure to adapt. Likewise for all cases of extinction, which represent well over 99 percent of species that ever lived. (This, by the way, poses an enormous problem for theories of intelligent design (ID). It doesn’t seem so intelligent to design millions of species that are destined to go extinct, and then replace them with other, similar species, most of which will also vanish. ID supporters have never addressed this difficulty.)
Natural selection must also work with the design of an organism as a whole, which is a compromise among different adaptations. Female sea turtles dig their nests on the beach with their flippers—a painful, slow, and clumsy process that exposes their eggs to predators. Having more shovel-like flippers would help them do a better and faster job, but then they couldn’t swim as well. A conscientious designer might have given the turtles an extra pair of limbs, with retractable shovel-like appendages, but turtles, like all reptiles, are stuck with a developmental plan that limits their limbs to four.