Two NASA satellites were critical for understanding the short bursts. The High-Energy Transient Explorer-2 (HETE-2) and Swift missions detected dozens of short GRBs. Using these observations, astronomers were able to craft the idea that a short GRB can occur when two dense neutron stars merge. A neutron star forms when the core of a star going supernova is not quite massive enough to form a black hole. In many cases, massive stars form in pairs, with the two stars orbiting one another, and many such high-mass star pairs are seen in our galaxy. Over time, the more massive star will explode, leaving behind a neutron star. Some time later, the other star explodes,
also
leaving behind a neutron star.
Through many forces, over billions of years, the orbits of the two stars will shrink. The two ultradense objects spiral closer and closer together . . . and then, finally, they will get so close that they literally merge. Their combined mass may be enough to form a black hole, and if enough matter is left over it will form an accretion disk around the hole. At this point, events are similar to what happens in the core of the massive star when it explodes: the accretion disk, tremendous magnetic fields, and powerful gravity of the black hole focus twin beams that explode outward.
Models of these events indicate that the burst of gamma rays would be much shorter in duration than the massive star type of GRB, and would produce higher-energy gamma rays. Both of these predictions fit the observations. There are other models that also fit the observations (such as a black hole-neutron star binary, with similar results), but this is the leading theory.
One major difference between the merging neutron star GRBs and the massive star hypernova GRBs is the time it takes before one can go off: while in modern times we expect to see few if any massive star GRBs, we expect to see plenty of neutron star mergers. It takes billions of years for the orbits of the two neutron stars to decay and cause the stars to merge, and so they should be able to occur today. This may very well be true, but in raw numbers they are less common than their more massive counterparts. This may be due to their uncommon origin—there are plenty more single massive stars that can explode than there are
binary
massive stars—so it’s difficult to get a handle on how many potential short GRBs there are in our galaxy. There are many neutron star binaries known, all of which could become short, hard GRBs . . . in a few more billion years. None are known that could go off in a century or millennium, or even in the next million years. But unlike massive stars, which are incredibly bright and obvious, binary neutron stars give off very little light and are difficult to detect.
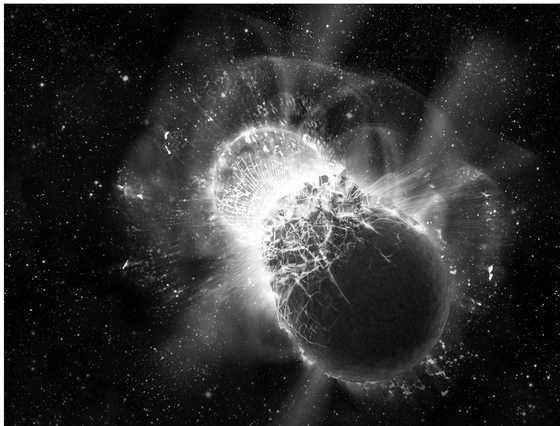
Two neutron stars finally succumb to their mutual gravity after billions of years of orbiting each other. Torn apart, they merge and collapse into a black hole, which announces its birth with a GRB.
DANA BERRY, SKYWORKS DIGITAL INC.
It’s unlikely in the extreme that there are any close enough to do us any harm. But it’s not possible to entirely rule them out either.
THE FUTURE IS BRIGHT
What we need, as always, are more observations. As the biggest explosions we know of—and probably the biggest explosions the Universe can make—GRBs are of great scientific interest. They tell us so much about how matter and energy act at the extreme limits of physics, how black holes are born and behave, and also about the environment around them. There’s still a lot we don’t understand about GRBs, of course. We’ve come a long way since the Vela satellites; in 2004, NASA launched the Swift satellite—so critical in understanding the origin of the short, hard bursts—which has observed hundreds of GRBs, including the most distant one ever seen at 12.8 billion light-years away. Swift’s observations have allowed keen insight into both long and short bursts, adding much-needed data to the theoretical models.
As we learn more about GRBs, we’ll be better able to assess the danger from them, including how they may have affected life on Earth in the past. While there’s probably nothing we can do if one goes off—and it’s incredibly unlikely it will happen at all—it’s always better to have a good handle on the situation.
So should you worry? I’m asked this all the time, and I have a simple answer: I have known people who’ve been killed in all sorts of unlikely ways, including car crashes and one who was hit by lightning. How many people do you know who have been killed by a gamma-ray burst?
CHAPTER 5
The Bottomless Pits of Black Holes
EVER VIGILANT, IT’S AN AMATEUR ASTRONOMER WHO
catches the first whiff of trouble.
He had hopes of doing some imaging of Uranus using his automated telescope, but the computer consistently points the telescope in the wrong direction. After going to manual, he eventually finds the giant planet several arc minutes from its calculated position. Puzzled, he calls a friend who quickly confirms that he has the same problem. An Internet search on a few astronomy bulletin boards reveals many such events from astronomers all over the world.
As days go by, things get worse. Jupiter seems to be off-kilter as well. Saturn, however, located on the other side of the Sun, appears unaffected. Rumors start to spread.
Then the situation gets really weird. The Solar and Heliospheric Observatory, parked in an orbit where the Earth’s gravity and the Sun’s gravity are in balance, starts to drift. Engineers are puzzled, but soon have other problems with which they must contend. Now Mars is in the wrong place. NASA has a probe on its way to the Red Planet; will it miss? But soon that point becomes moot, as the spacecraft is drifting too. After a few days it’s clear the probe is lost . . . and that the probe is the least of our worries.
Solar astronomers detect that the Sun’s position is off as well. That doesn’t make
any
sense. What could move an entire star . . . ? But they quickly realize the trouble is not with the Sun, but with the
Earth.
Like the other planets, the Earth is no longer circling the Sun as usual, but is moving off its prescribed orbit.
Panic spreads. Scientists come to the obvious conclusion: some massive object is approaching the Earth, and its gravity is pulling us off course. They use the data on the other planets’ motions to determine where this object must be, but find nothing at that location of the sky.
Ironically, seeing nothing confirms their worst fears: it’s a black hole. Backtracking its position reveals it’s headed almost straight at us at the incredible speed of 500 miles per second. Astronomers calculate its mass as a terrifying ten times that of the Sun’s—easily enough to spell doom for us on Earth. The gravitational effects are subtle at first, but accelerate.
Just a few weeks after the first trouble began—and its position still 300 million miles away—the black hole’s gravity as felt on Earth is equal to that of the Sun. Earth no longer orbits one star: it is enthralled by two: one living, one dead. Within a few more days, the black hole’s influence is far stronger than the Sun’s. Grasping the Earth with invisible fingers, it tears us away from the Sun, bringing us closer to the collapsed star.
As we approach, the gravitational tides from the black hole begin to stretch the Earth. Tides from the Moon cause the oceans to ebb and flow, but the black hole has 200 million times the mass of the Moon. Even from millions of miles away, the tidal force is causing enormous floods, gigantic earthquakes, tsunamis.
The coup de grâce quickly arrives. When the black hole reaches a distance of just seven million miles, the force of its gravity as felt by objects on the Earth’s surface is equal to the gravity of the Earth itself. The few survivors of the past few days’ events suddenly find themselves weightless as they are pulled both up and down with equal force.
Within minutes, as the black hole draws ever closer, the force upward dominates. A rising hurricane of air now blows weightless people up, along with rocks, cars, the oceans . . .
An hour later, it’s all over. The immense gravity of the dead star rips the Earth to pieces, shredding it into vapor. The material that once constituted our home world falls toward the voracious maw of the hole, swirling around it ever faster, forming a disk of million-degree plasma before taking the final plunge.
Without a hiccup, without a stumble, the black hole sails on, down and out of the solar system, leaving behind chaos, scattered planets, and death.
THE HOLE TRUTH
What is it about black holes? The mind-boggling physics, the sheer destructive power, the weird way they twist our notions of reality, space, and time?
Maybe they fascinate us simply because they’re
cool.
Born in the hellish heart of a supernova, announcing their presence with twin beams of unstoppable fury, and devouring (almost) all that is in their path, black holes are firmly fixed in the public’s mind. Movies, television shows, books, countless articles, and endless discussion have revolved around them. Yet with all this excitement and interest, most people really have only a vague idea of just what black holes are, and what they can and cannot do.
But never forget, they’re
dangerous.
There are many ways a black hole can kill you. Some are simple, and some are truly bizarre. Unless you’re looking for trouble, they’re all unlikely in the extreme, but if you want rampant destruction on a large scale, then a black hole is a good place to start.
I’VE FALLEN AND I CAN’T GET UP
As pointed out in chapter 4, a black hole, by definition, is an object whose escape velocity is equal to or greater than the speed of light. That means that anything that falls in cannot get out, because as far as we know nothing can exceed the speed of light.
Therefore, the first and most obvious danger from a black hole is, simply, falling in. If that happens, well, that’s that. It’s a one-way trip. You’re done. End of discussion.
As a way a black hole can kill you, that’s not terribly exciting—no death rays, no vast and terrible wreaking of havoc. Just bloop! And you’re gone.
This lack of drama is a bit unsatisfying from a storytelling stance. But it also defies our common sense.
36
If you’re in a rocket plunging into a black hole, can’t you just turn the rocket around and thrust really, really hard and get out?
No, you can’t. The extremely strong gravity near a black hole forces us to change the way we think about space, time, and motion.
Mathematically, the gravitational pull you feel from an object drops as the square of your distance from that object; double your distance from an object and the gravity you feel from it drops by a factor of 2 × 2 = 4. Get ten times farther away and the force drops by 10 × 10 = 100. Make the distance as big as you please; gravity goes on forever, and the force never actually drops to zero.
37
So imagine you are on the surface of the Earth (which should be easy enough to do) and you have a ball in your hand. You throw it straight up into the air. As it goes up, gravity pulls on it, slowing its velocity. Eventually, the ball stops (velocity = 0) and then starts to fall back to Earth, accelerating the whole way down until you catch it.
Now imagine you throw the ball very high, like several miles high. Gravity pulls it downward as it goes up, slowing it, but as it gets higher up,
the force of gravity is getting weaker because it’s farther from the Earth.
So it’s slowing down, but as it gets higher, the
rate
at which it’s slowing is
itself
slowing, because gravity is getting weaker with height.
This means that if you can throw the ball at just the right speed, gravity will slow it down at the same rate that gravity itself is getting weaker. The ball will always slow down, but never actually reach zero. It will
always
move away from the Earth, but ever more slowly.
That’s the definition of
escape velocity
—the initial velocity you have to give a projectile such that it will always move away from an object (like the Earth), always slowing down, but never stopping, and never falling back.
If you throw a ball up with slightly less than escape velocity, it will go a long way, but it will eventually come back. If you throw it harder, it’ll just go away. At escape velocity—seven miles per second for the surface of the Earth—the ball is just able to escape from the Earth.
However, since gravity gets weaker with distance, the escape velocity gets smaller with distance too. If you were on top of a very tall mountain, the velocity at which you have to throw a ball is slightly less than the velocity you’d have to give it down at sea level. Also, escape velocity is an impulse; that is, it’s the velocity you have to give an object all at once to get it to escape. If you can somehow continue to add velocity to a projectile as it heads up, then the concept of escape velocity gets a little trickier.